Programming Language Simplifies Design of Biological Circuits
By LabMedica International staff writers Posted on 05 Apr 2016 |
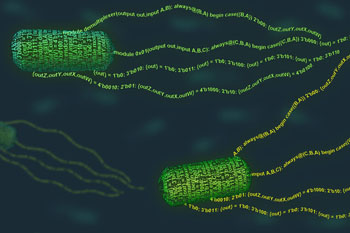
Image: Using E. coli bacteria as the initial organism for development, bioengineers have devised a programming language that simplifies the process of designing and incorporating synthetic gene elements to provide living cells with new functions (Photo courtesy of Janet Iwasa and MIT).
Bioengineers have created a programming language that allows to rapidly design complex DNA-encoded circuits that provide new functions to living cells. In a first application, genetic circuits generated on plasmids expressed in Escherichia coli bacteria successfully regulated cellular functions in response to multiple environmental signals.
The word was led by researchers at Massachusetts Institute of Technology (Cambridge, MA, USA) in collaboration with researchers at Boston University (Boston, MA, USA) and National Institute of Standards and Technology (Gaithersburg, MD, USA). They applied principles from electronic design automation (EDA) to simplify the process of incorporating synthetic gene regulation into cells and to enable increased circuit complexity.
“It is literally a programming language for bacteria,” says Christopher Voigt, professor, MIT, “You use a text-based language, just like you’re programming a computer. Then you take that text and you compile it and it turns it into a DNA sequence that you put into the cell, and the circuit runs inside the cell.”
Over the past 15 years, scientists have designed many genetic parts (e.g., sensors, memory switches, biological clocks) that can be combined to modify existing cell functions and add new ones. However, designing each circuit is a laborious process that requires expertise and often much trial and error. “You have to have this really intimate knowledge of how those pieces are going to work,” said Prof. Voigt. Users of the new programming language, however, need no special knowledge of genetic engineering. “You could be completely naive as to how any of it works. That’s what’s really different about this,” he said, “You could be a student in high school and go onto the Web-based server and type out the program you want, and it spits back the DNA sequence.”
The language is based on the hardware description language Verilog commonly used to program computer chips. To create a version that would work for cells, the researchers designed computing elements (such as logic gates and sensors) that can be encoded in DNA. The sensors can detect different compounds, such as oxygen or glucose, as well as light, temperature, acidity, and other environmental conditions. Users can also add their own sensors. “It’s very customizable,” said Prof. Voigt.
Another advantage of this technique is its speed. Until now, “it would take years to build these types of circuits. Now you just hit the button and immediately get a DNA sequence to test,” said Prof. Voigt. This approach simplifies incorporation of genetic circuits for decision-making, control, sensing, or spatial organization. The user specifies the desired circuit function in Verilog code, and this is automatically transformed into a DNA sequence. Algorithms build a circuit diagram, assign and connect gates, and simulate performance.
In the study, out of 60 circuits programmed for different functions, 45 worked correctly the first time tested. Many of the circuits were designed to measure and respond to one or more environmental conditions, such as oxygen level or glucose concentration. Another circuit was designed to rank three different inputs and then respond based on the priority of each.
In the current version, the genetic parts are optimized for E. coli. The researchers are expanding the language for other strains of bacteria, as well as the yeast Saccharomyces cerevisiae. This would allow users to write a single program and then compile it for different organisms to get the right DNA sequence for each.
The team plans to develop applications such as bacteria that can be swallowed to aid in digestion of lactose; bacteria that can live on plant roots and produce insecticide if they sense the plant is under attack; bacteria that can produce a cancer drug upon detecting a tumor; and yeast cells that can halt their own fermentation process if too many toxic byproducts build up in a reactor.
The study, by Nielsen AAK et al, was published April 1, 2016, in the journal Science.
Related Links:
Massachusetts Institute of Technology
The word was led by researchers at Massachusetts Institute of Technology (Cambridge, MA, USA) in collaboration with researchers at Boston University (Boston, MA, USA) and National Institute of Standards and Technology (Gaithersburg, MD, USA). They applied principles from electronic design automation (EDA) to simplify the process of incorporating synthetic gene regulation into cells and to enable increased circuit complexity.
“It is literally a programming language for bacteria,” says Christopher Voigt, professor, MIT, “You use a text-based language, just like you’re programming a computer. Then you take that text and you compile it and it turns it into a DNA sequence that you put into the cell, and the circuit runs inside the cell.”
Over the past 15 years, scientists have designed many genetic parts (e.g., sensors, memory switches, biological clocks) that can be combined to modify existing cell functions and add new ones. However, designing each circuit is a laborious process that requires expertise and often much trial and error. “You have to have this really intimate knowledge of how those pieces are going to work,” said Prof. Voigt. Users of the new programming language, however, need no special knowledge of genetic engineering. “You could be completely naive as to how any of it works. That’s what’s really different about this,” he said, “You could be a student in high school and go onto the Web-based server and type out the program you want, and it spits back the DNA sequence.”
The language is based on the hardware description language Verilog commonly used to program computer chips. To create a version that would work for cells, the researchers designed computing elements (such as logic gates and sensors) that can be encoded in DNA. The sensors can detect different compounds, such as oxygen or glucose, as well as light, temperature, acidity, and other environmental conditions. Users can also add their own sensors. “It’s very customizable,” said Prof. Voigt.
Another advantage of this technique is its speed. Until now, “it would take years to build these types of circuits. Now you just hit the button and immediately get a DNA sequence to test,” said Prof. Voigt. This approach simplifies incorporation of genetic circuits for decision-making, control, sensing, or spatial organization. The user specifies the desired circuit function in Verilog code, and this is automatically transformed into a DNA sequence. Algorithms build a circuit diagram, assign and connect gates, and simulate performance.
In the study, out of 60 circuits programmed for different functions, 45 worked correctly the first time tested. Many of the circuits were designed to measure and respond to one or more environmental conditions, such as oxygen level or glucose concentration. Another circuit was designed to rank three different inputs and then respond based on the priority of each.
In the current version, the genetic parts are optimized for E. coli. The researchers are expanding the language for other strains of bacteria, as well as the yeast Saccharomyces cerevisiae. This would allow users to write a single program and then compile it for different organisms to get the right DNA sequence for each.
The team plans to develop applications such as bacteria that can be swallowed to aid in digestion of lactose; bacteria that can live on plant roots and produce insecticide if they sense the plant is under attack; bacteria that can produce a cancer drug upon detecting a tumor; and yeast cells that can halt their own fermentation process if too many toxic byproducts build up in a reactor.
The study, by Nielsen AAK et al, was published April 1, 2016, in the journal Science.
Related Links:
Massachusetts Institute of Technology
Latest BioResearch News
- Genome Analysis Predicts Likelihood of Neurodisability in Oxygen-Deprived Newborns
- Gene Panel Predicts Disease Progession for Patients with B-cell Lymphoma
- New Method Simplifies Preparation of Tumor Genomic DNA Libraries
- New Tool Developed for Diagnosis of Chronic HBV Infection
- Panel of Genetic Loci Accurately Predicts Risk of Developing Gout
- Disrupted TGFB Signaling Linked to Increased Cancer-Related Bacteria
- Gene Fusion Protein Proposed as Prostate Cancer Biomarker
- NIV Test to Diagnose and Monitor Vascular Complications in Diabetes
- Semen Exosome MicroRNA Proves Biomarker for Prostate Cancer
- Genetic Loci Link Plasma Lipid Levels to CVD Risk
- Newly Identified Gene Network Aids in Early Diagnosis of Autism Spectrum Disorder
- Link Confirmed between Living in Poverty and Developing Diseases
- Genomic Study Identifies Kidney Disease Loci in Type I Diabetes Patients
- Liquid Biopsy More Effective for Analyzing Tumor Drug Resistance Mutations
- New Liquid Biopsy Assay Reveals Host-Pathogen Interactions
- Method Developed for Enriching Trophoblast Population in Samples
Channels
Clinical Chemistry
view channel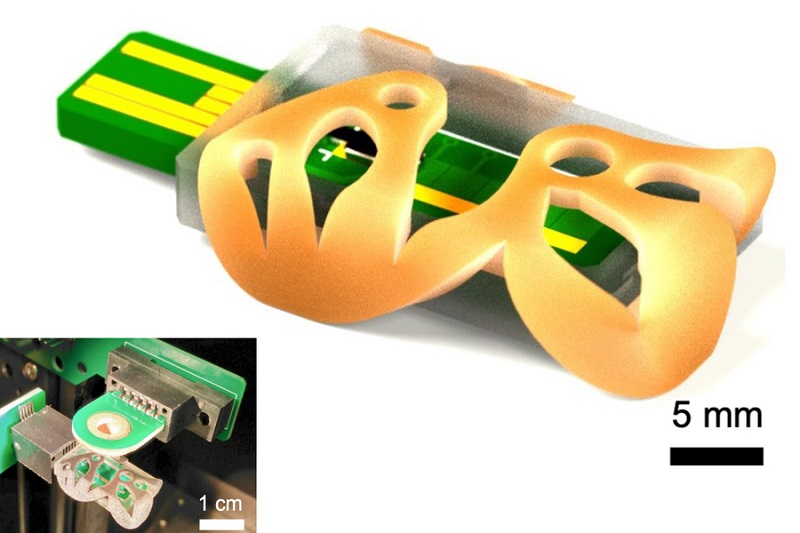
3D Printed Point-Of-Care Mass Spectrometer Outperforms State-Of-The-Art Models
Mass spectrometry is a precise technique for identifying the chemical components of a sample and has significant potential for monitoring chronic illness health states, such as measuring hormone levels... Read more.jpg)
POC Biomedical Test Spins Water Droplet Using Sound Waves for Cancer Detection
Exosomes, tiny cellular bioparticles carrying a specific set of proteins, lipids, and genetic materials, play a crucial role in cell communication and hold promise for non-invasive diagnostics.... Read more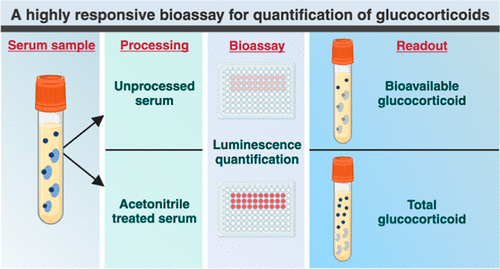
Highly Reliable Cell-Based Assay Enables Accurate Diagnosis of Endocrine Diseases
The conventional methods for measuring free cortisol, the body's stress hormone, from blood or saliva are quite demanding and require sample processing. The most common method, therefore, involves collecting... Read moreMolecular Diagnostics
view channel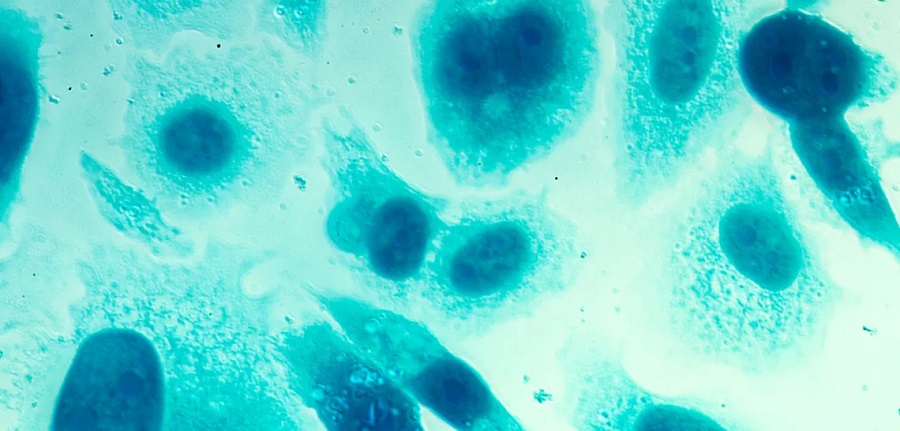
Blood Proteins Could Warn of Cancer Seven Years before Diagnosis
Two studies have identified proteins in the blood that could potentially alert individuals to the presence of cancer more than seven years before the disease is clinically diagnosed. Researchers found... Read more (1)_1.jpg)
Ultrasound-Aided Blood Testing Detects Cancer Biomarkers from Cells
Ultrasound imaging serves as a noninvasive method to locate and monitor cancerous tumors effectively. However, crucial details about the cancer, such as the specific types of cells and genetic mutations... Read moreHematology
view channel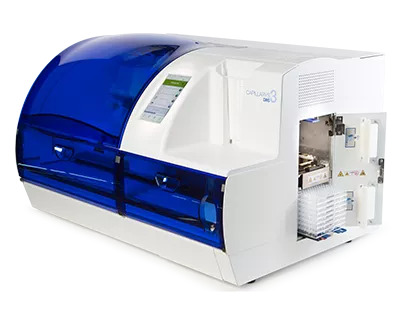
Next Generation Instrument Screens for Hemoglobin Disorders in Newborns
Hemoglobinopathies, the most widespread inherited conditions globally, affect about 7% of the population as carriers, with 2.7% of newborns being born with these conditions. The spectrum of clinical manifestations... Read more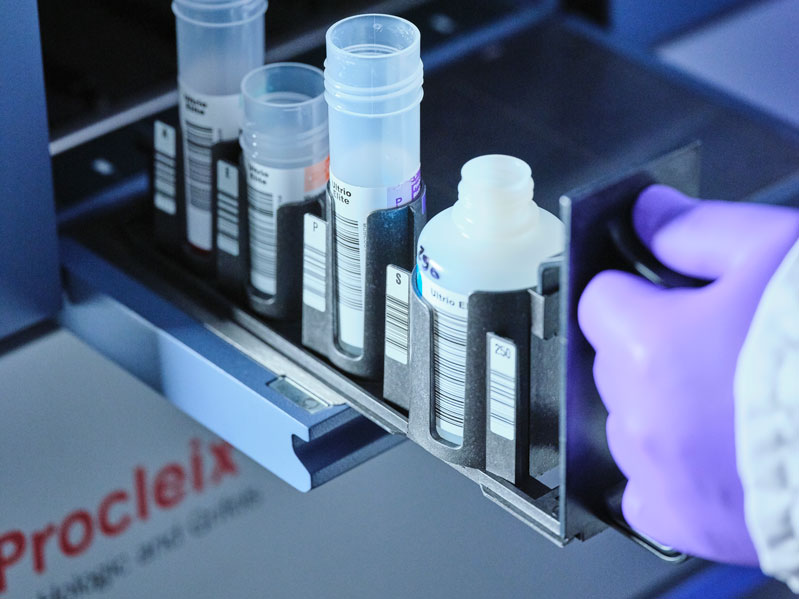
First 4-in-1 Nucleic Acid Test for Arbovirus Screening to Reduce Risk of Transfusion-Transmitted Infections
Arboviruses represent an emerging global health threat, exacerbated by climate change and increased international travel that is facilitating their spread across new regions. Chikungunya, dengue, West... Read more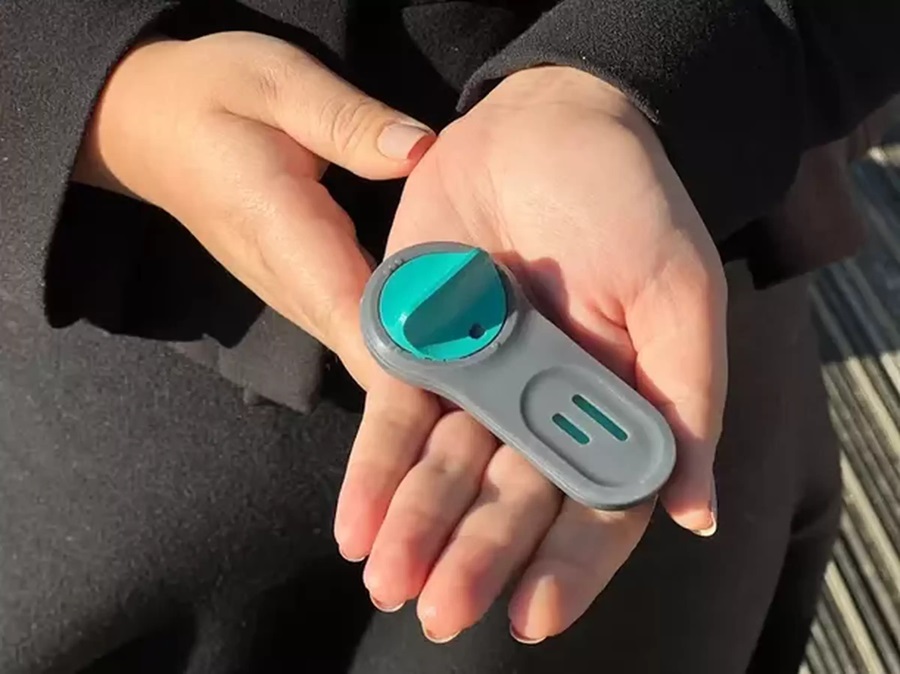
POC Finger-Prick Blood Test Determines Risk of Neutropenic Sepsis in Patients Undergoing Chemotherapy
Neutropenia, a decrease in neutrophils (a type of white blood cell crucial for fighting infections), is a frequent side effect of certain cancer treatments. This condition elevates the risk of infections,... Read more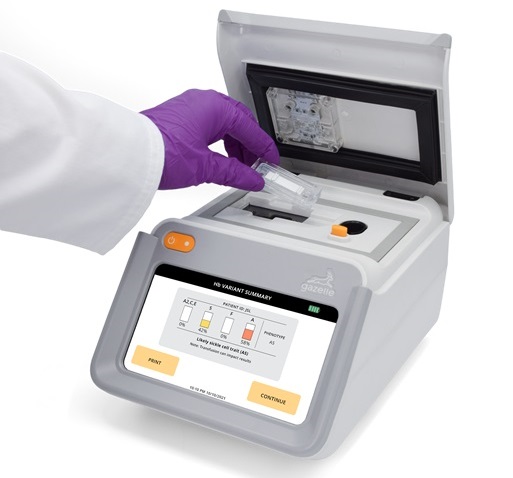
First Affordable and Rapid Test for Beta Thalassemia Demonstrates 99% Diagnostic Accuracy
Hemoglobin disorders rank as some of the most prevalent monogenic diseases globally. Among various hemoglobin disorders, beta thalassemia, a hereditary blood disorder, affects about 1.5% of the world's... Read moreImmunology
view channel.jpg)
AI Predicts Tumor-Killing Cells with High Accuracy
Cellular immunotherapy involves extracting immune cells from a patient's tumor, potentially enhancing their cancer-fighting capabilities through engineering, and then expanding and reintroducing them into the body.... Read more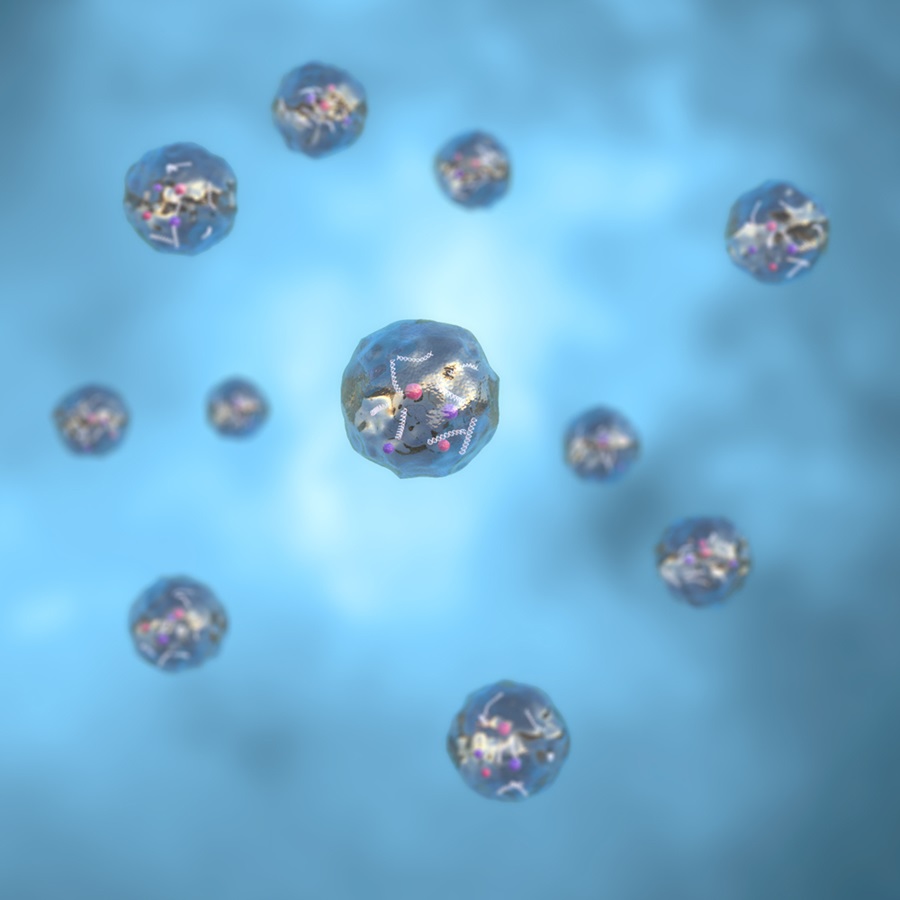
Diagnostic Blood Test for Cellular Rejection after Organ Transplant Could Replace Surgical Biopsies
Transplanted organs constantly face the risk of being rejected by the recipient's immune system which differentiates self from non-self using T cells and B cells. T cells are commonly associated with acute... Read more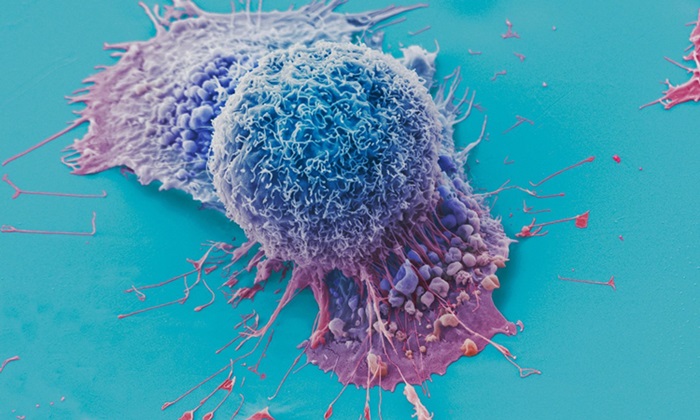
AI Tool Precisely Matches Cancer Drugs to Patients Using Information from Each Tumor Cell
Current strategies for matching cancer patients with specific treatments often depend on bulk sequencing of tumor DNA and RNA, which provides an average profile from all cells within a tumor sample.... Read more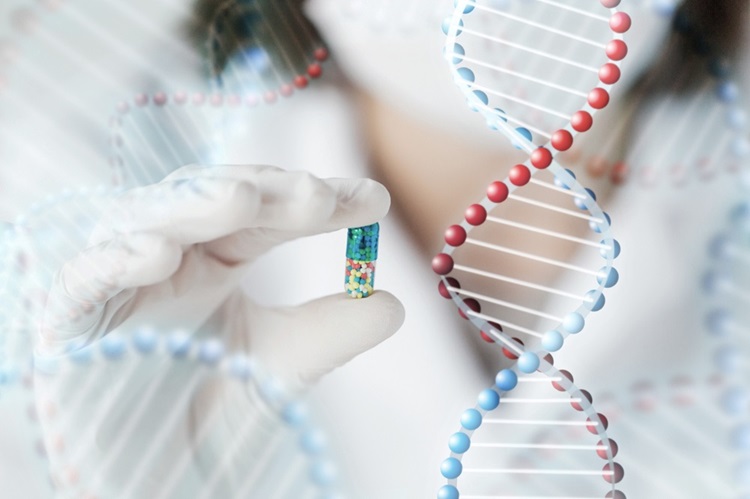
Genetic Testing Combined With Personalized Drug Screening On Tumor Samples to Revolutionize Cancer Treatment
Cancer treatment typically adheres to a standard of care—established, statistically validated regimens that are effective for the majority of patients. However, the disease’s inherent variability means... Read moreMicrobiology
view channel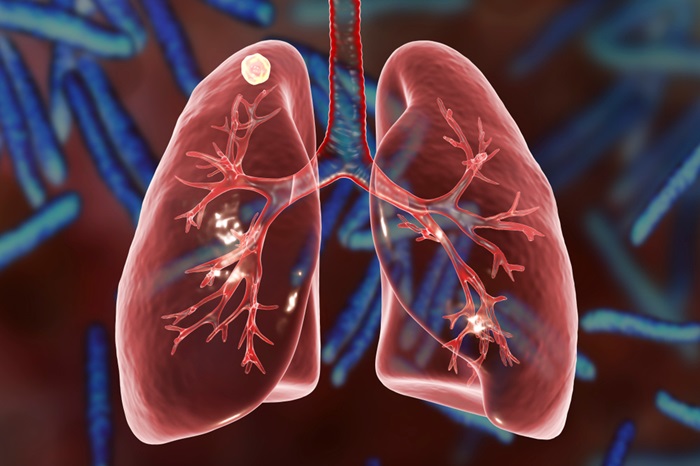
Integrated Solution Ushers New Era of Automated Tuberculosis Testing
Tuberculosis (TB) is responsible for 1.3 million deaths every year, positioning it as one of the top killers globally due to a single infectious agent. In 2022, around 10.6 million people were diagnosed... Read more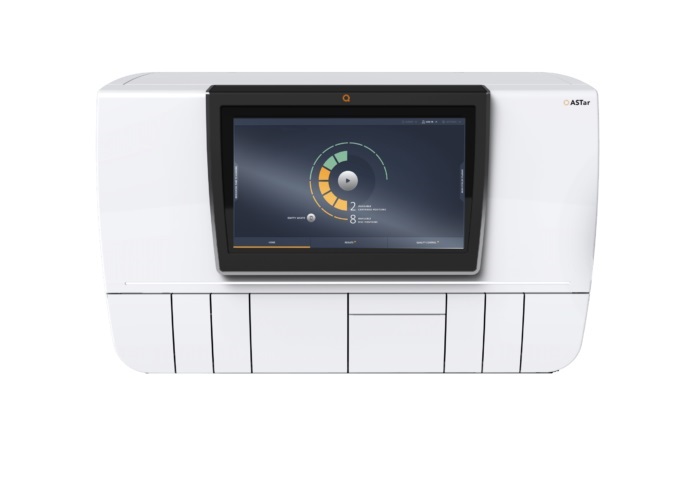
Automated Sepsis Test System Enables Rapid Diagnosis for Patients with Severe Bloodstream Infections
Sepsis affects up to 50 million people globally each year, with bacteraemia, formerly known as blood poisoning, being a major cause. In the United States alone, approximately two million individuals are... Read more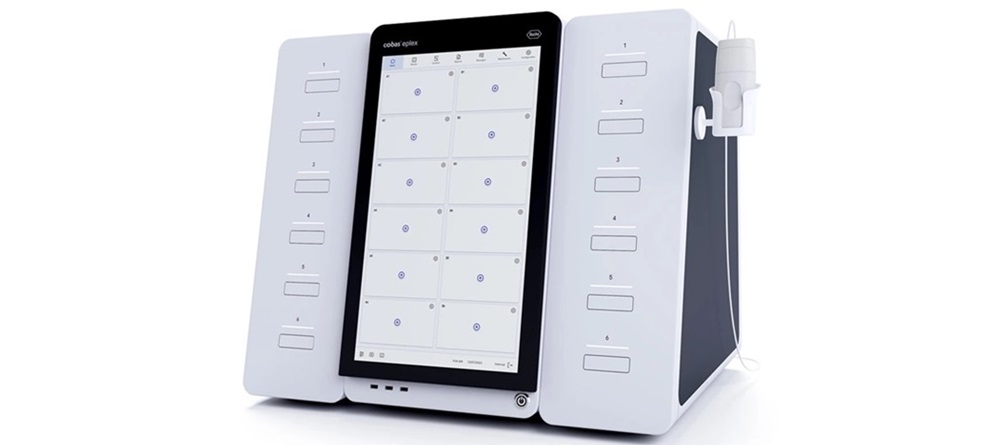
Enhanced Rapid Syndromic Molecular Diagnostic Solution Detects Broad Range of Infectious Diseases
GenMark Diagnostics (Carlsbad, CA, USA), a member of the Roche Group (Basel, Switzerland), has rebranded its ePlex® system as the cobas eplex system. This rebranding under the globally renowned cobas name... Read more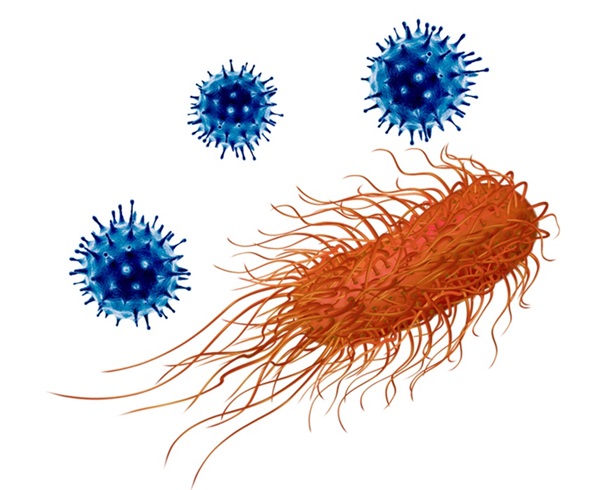
Clinical Decision Support Software a Game-Changer in Antimicrobial Resistance Battle
Antimicrobial resistance (AMR) is a serious global public health concern that claims millions of lives every year. It primarily results from the inappropriate and excessive use of antibiotics, which reduces... Read morePathology
view channel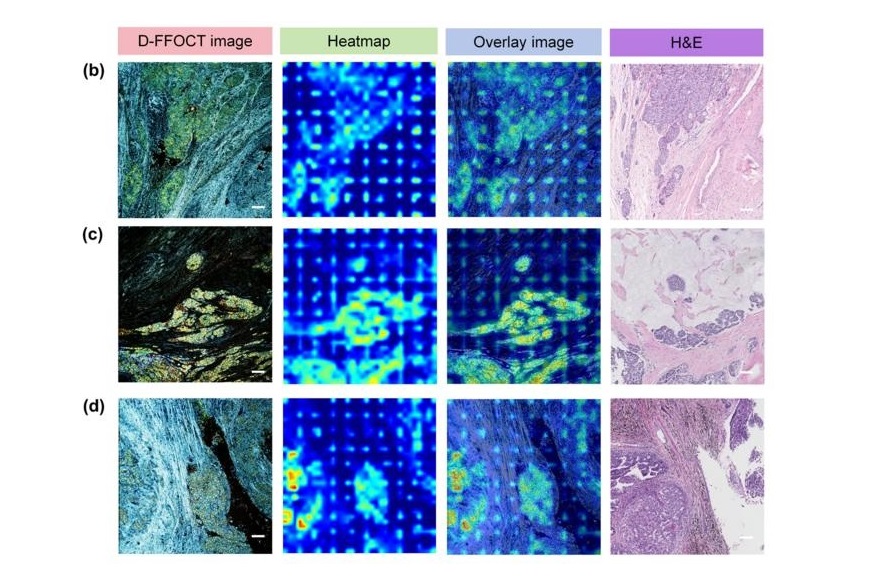
AI Integrated With Optical Imaging Technology Enables Rapid Intraoperative Diagnosis
Rapid and accurate intraoperative diagnosis is essential for tumor surgery as it guides surgical decisions with precision. Traditional intraoperative assessments, such as frozen sections based on H&E... Read more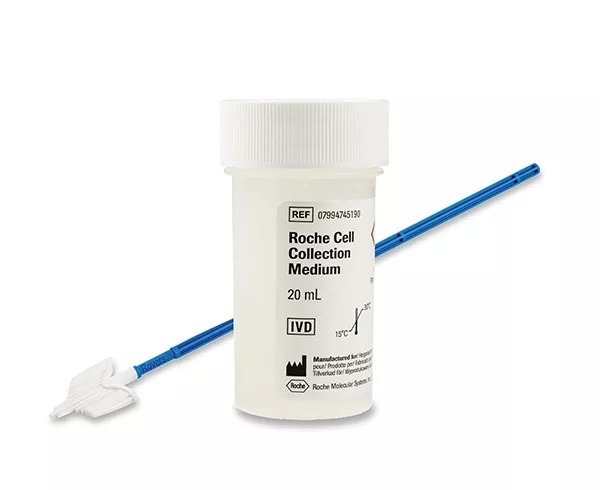
HPV Self-Collection Solution Improves Access to Cervical Cancer Testing
Annually, over 604,000 women across the world are diagnosed with cervical cancer, and about 342,000 die from this disease, which is preventable and primarily caused by the Human Papillomavirus (HPV).... Read more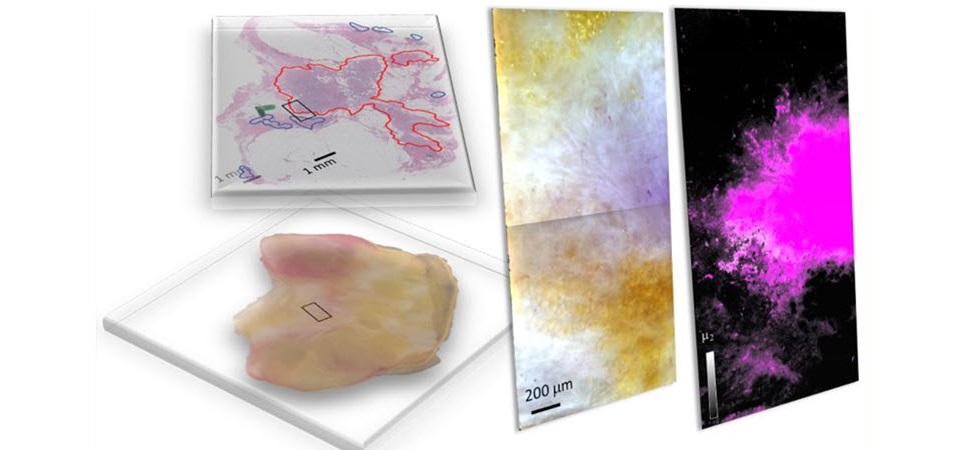
Hyperspectral Dark-Field Microscopy Enables Rapid and Accurate Identification of Cancerous Tissues
Breast cancer remains a major cause of cancer-related mortality among women. Breast-conserving surgery (BCS), also known as lumpectomy, is the removal of the cancerous lump and a small margin of surrounding tissue.... Read moreTechnology
view channel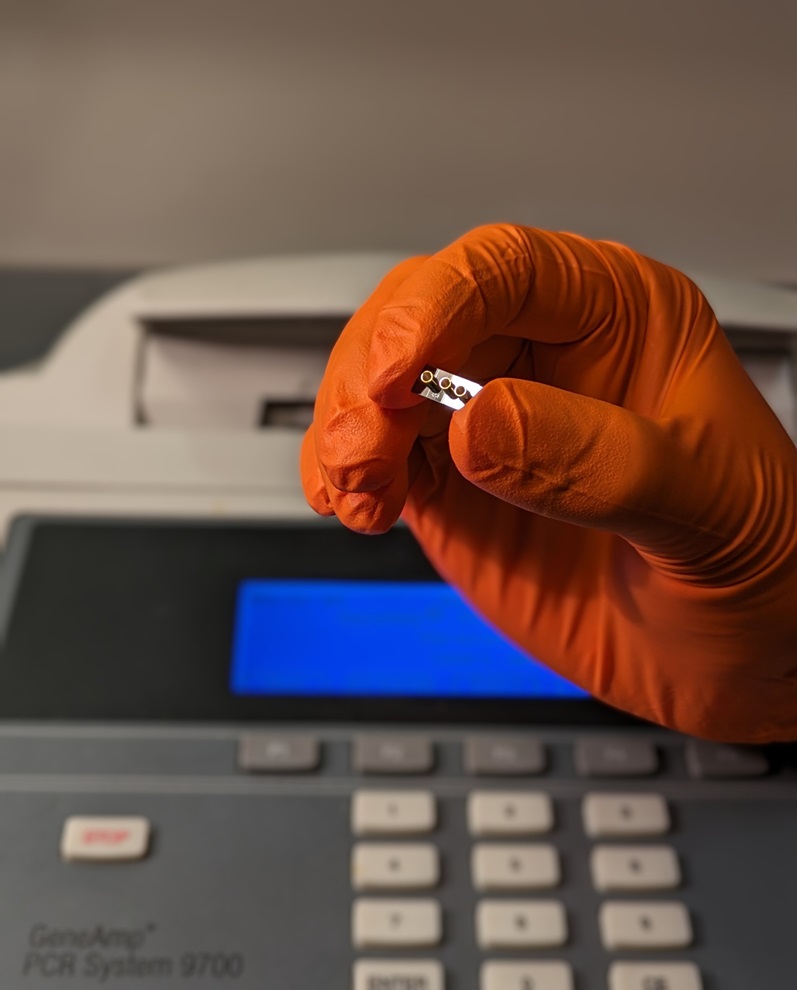
New Diagnostic System Achieves PCR Testing Accuracy
While PCR tests are the gold standard of accuracy for virology testing, they come with limitations such as complexity, the need for skilled lab operators, and longer result times. They also require complex... Read more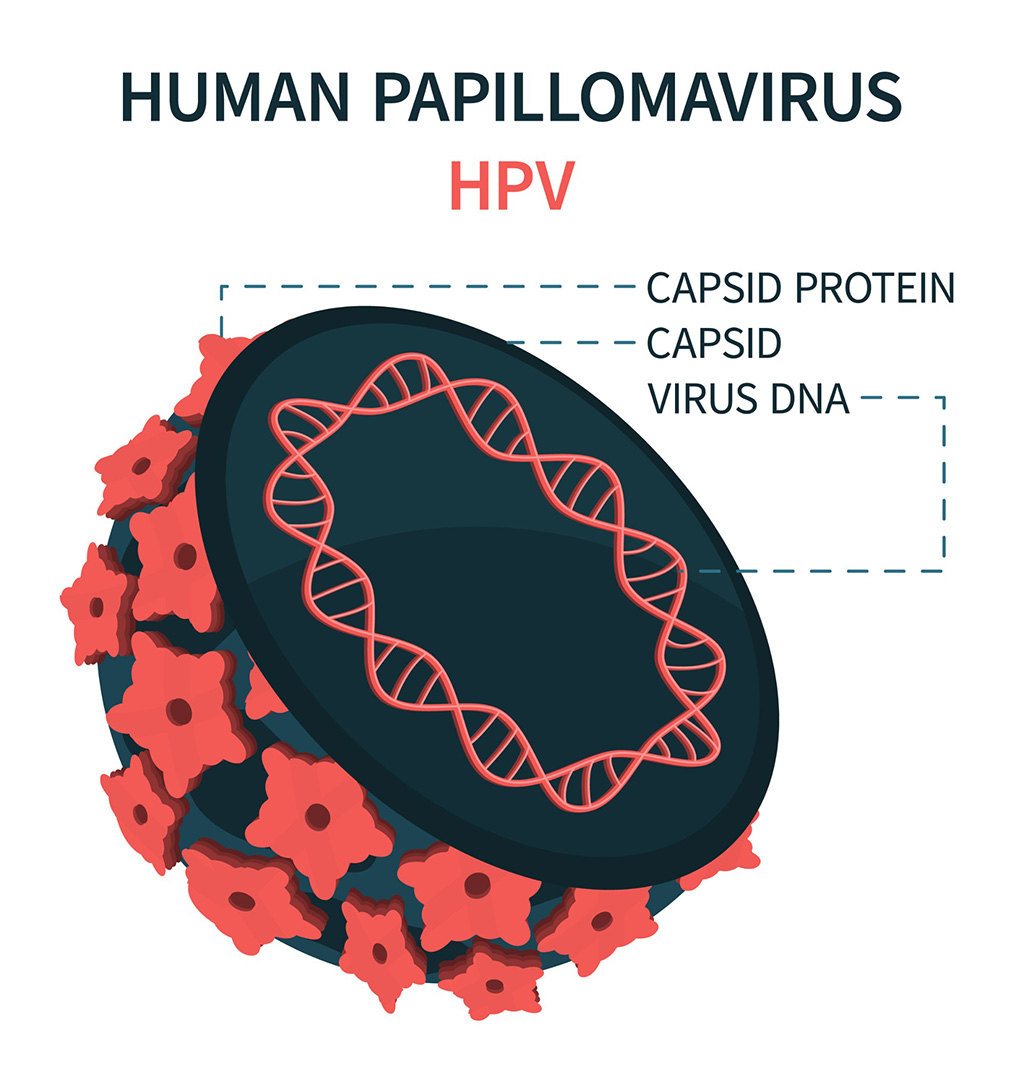
DNA Biosensor Enables Early Diagnosis of Cervical Cancer
Molybdenum disulfide (MoS2), recognized for its potential to form two-dimensional nanosheets like graphene, is a material that's increasingly catching the eye of the scientific community.... Read more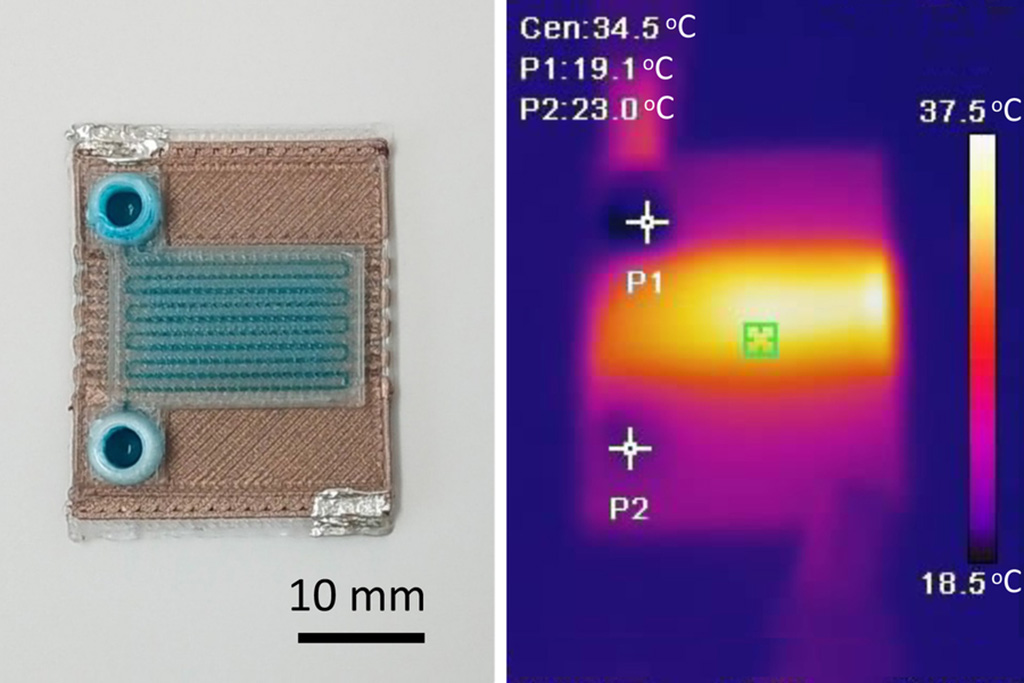
Self-Heating Microfluidic Devices Can Detect Diseases in Tiny Blood or Fluid Samples
Microfluidics, which are miniature devices that control the flow of liquids and facilitate chemical reactions, play a key role in disease detection from small samples of blood or other fluids.... Read more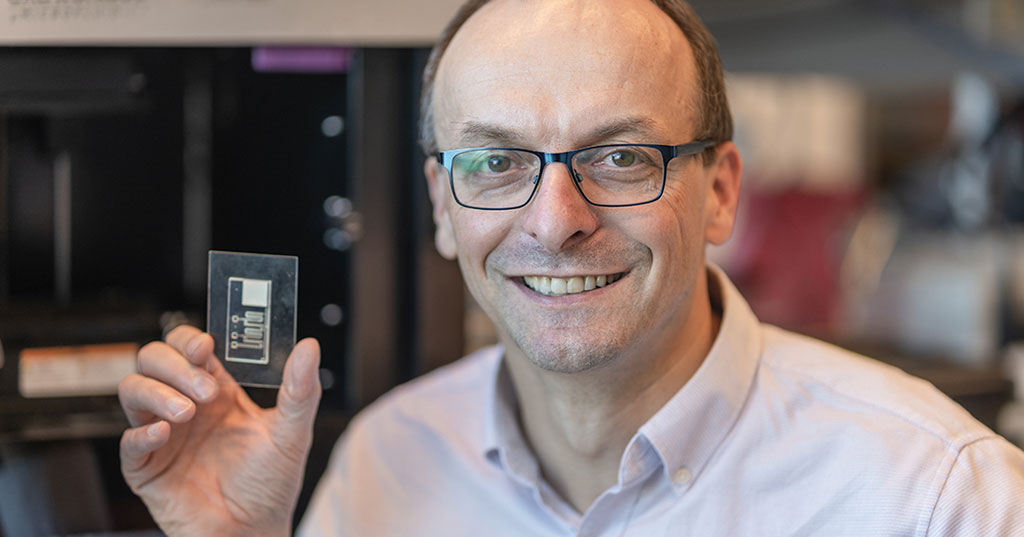
Breakthrough in Diagnostic Technology Could Make On-The-Spot Testing Widely Accessible
Home testing gained significant importance during the COVID-19 pandemic, yet the availability of rapid tests is limited, and most of them can only drive one liquid across the strip, leading to continued... Read moreIndustry
view channel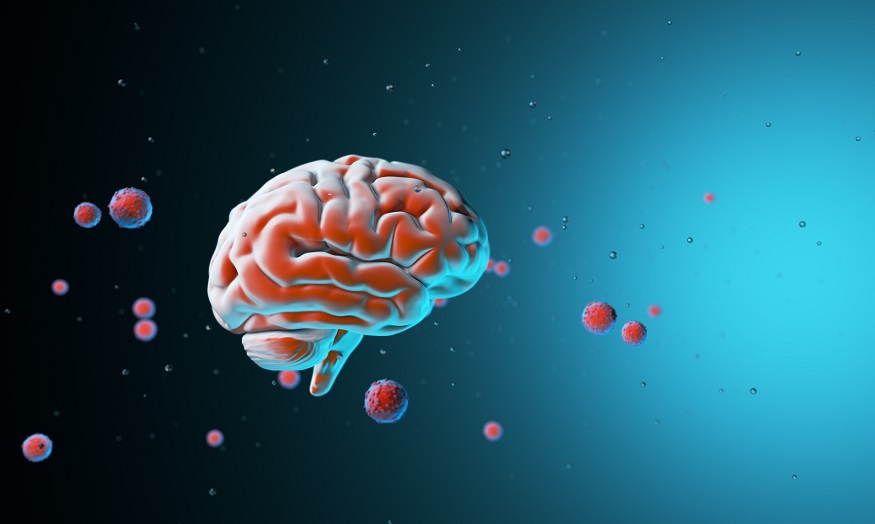
Danaher and Johns Hopkins University Collaborate to Improve Neurological Diagnosis
Unlike severe traumatic brain injury (TBI), mild TBI often does not show clear correlations with abnormalities detected through head computed tomography (CT) scans. Consequently, there is a pressing need... Read more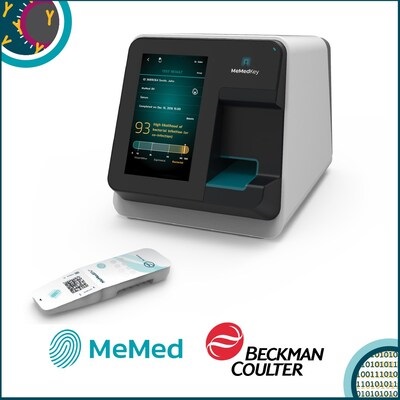
Beckman Coulter and MeMed Expand Host Immune Response Diagnostics Partnership
Beckman Coulter Diagnostics (Brea, CA, USA) and MeMed BV (Haifa, Israel) have expanded their host immune response diagnostics partnership. Beckman Coulter is now an authorized distributor of the MeMed... Read more_1.jpg)