Functional 3D Brain-Like Tissue Model Bioengineered
By LabMedica International staff writers
Posted on 20 Aug 2014
Researchers recently reported on the development of the first complex, three-dimensional (3D) model comprised of brain-like cortical tissue that displays biochemical and electrophysiologic responses, and can function in the laboratory for months. The engineered tissue model offers new approaches for examining brain function, disease, and trauma, and treatment. Posted on 20 Aug 2014
The US National Institutes of Health (NIH; Bethesda, MD, USA)-funded study findings were published in the August 11, 2014, of the Proceedings of the National Academy of Sciences of the United States of America (PNAS). Furthering the study of brain trauma, disease, and therapeutic treatments is something that the study’s senior and corresponding author, David Kaplan, PhD, is a professor and chair of biomedical engineering at Tufts School of Engineering (Medford/Somerville, MA, USA), has wanted to pursue for quite a while.
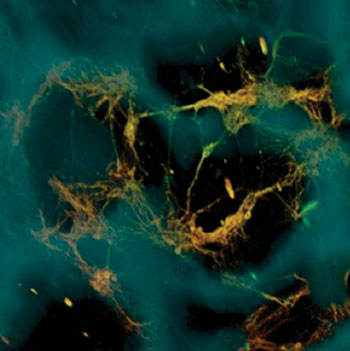
Image: Neurons (greenish yellow) attach to silk-based scaffold (blue) creating functional networks throughout the scaffold pores (dark areas) (Photo courtesy of Tufts University).
The human brain remains one of the least understood organs in the human body, because of its complexity and the difficulty of studying its physiology in the living body. “There are few good options for studying the physiology of the living brain, yet this is perhaps one of the biggest areas of unmet clinical need when you consider the need for new options to understand and treat a wide range of neurological disorders associated with the brain. To generate this system that has such great value is very exciting for our team,” said Dr. Kaplan, who directs the NIH-funded P41 Tissue Engineering Resource Center based at Tufts.
Instead of rebuilding a whole-brain network, the Tufts scientists generated a modular design that replicated basic features most pertinent to the brain’s tissue-level physiologic functions. Each module merged two substances with diverse characteristics: a stiffer porous scaffold comprised of cast silk protein on which the cortical neurons, derived from rats, could anchor and a softer collagen gel matrix that allowed axons to penetrate and connect in a three dimensional (3D) way. Circular modules of cast silk were punched into doughnuts, and then assembled into concentric rings to simulate the laminal layers of the neocortex. Each layer was seeded with neurons independently before assembly, without the need for adhesive or glue. The doughnuts were then immersed in the collagen gel matrix.
The silk-collagen gel combo provided an ideal microenvironment for neural network formation and function. “The stiffness of the silk biomaterial could be tuned to accommodate the cortical neurons and the different types of gels, maintaining both stability in culture and brain-like tissue elasticity,” said the study’s first author, Min D. Tang-Schomer, PhD, postdoctoral scholar in biomedical engineering at Tufts. “The tissue maintained viability for at least nine weeks—significantly longer than cultures made of collagen or hydrogel alone—and also offered structural support for network connectivity that is crucial for brain activity.”
The Tufts researchers were able to use the tissue model to examine multiple post-injury effects, including cellular damage, neurochemical changes, and electrophysiological activity. For example, when a weight was dropped on the model tissue to simulate a traumatic brain injury, the tissue released high levels of the chemical glutamate, a neurotransmitter known to be emitted by cells following brain damage; the tissue also showed transient electrical hyperactivity consistent with post-trauma responses observed in vivo.
“This model provides a unique opportunity for mapping out real-time neurophysiological events and function-studies in the laboratory, monitoring that is prohibitive with humans or animals,” said study coauthor Philip Haydon, PhD, a professor of neuroscience at the Sackler School of Graduate Biomedical Sciences, Tufts University School of Medicine.
According to Dr. Kaplan, this research is underway to additionally engineer the model. It could potentially be applied to study brain structure-function, drug screening, impact of electrodes and implants on brain function, disease formation and treatments, and the effects of nutrition and toxicants. “This is the first step,” he emphasized.
Related Links:
Tufts School of Engineering